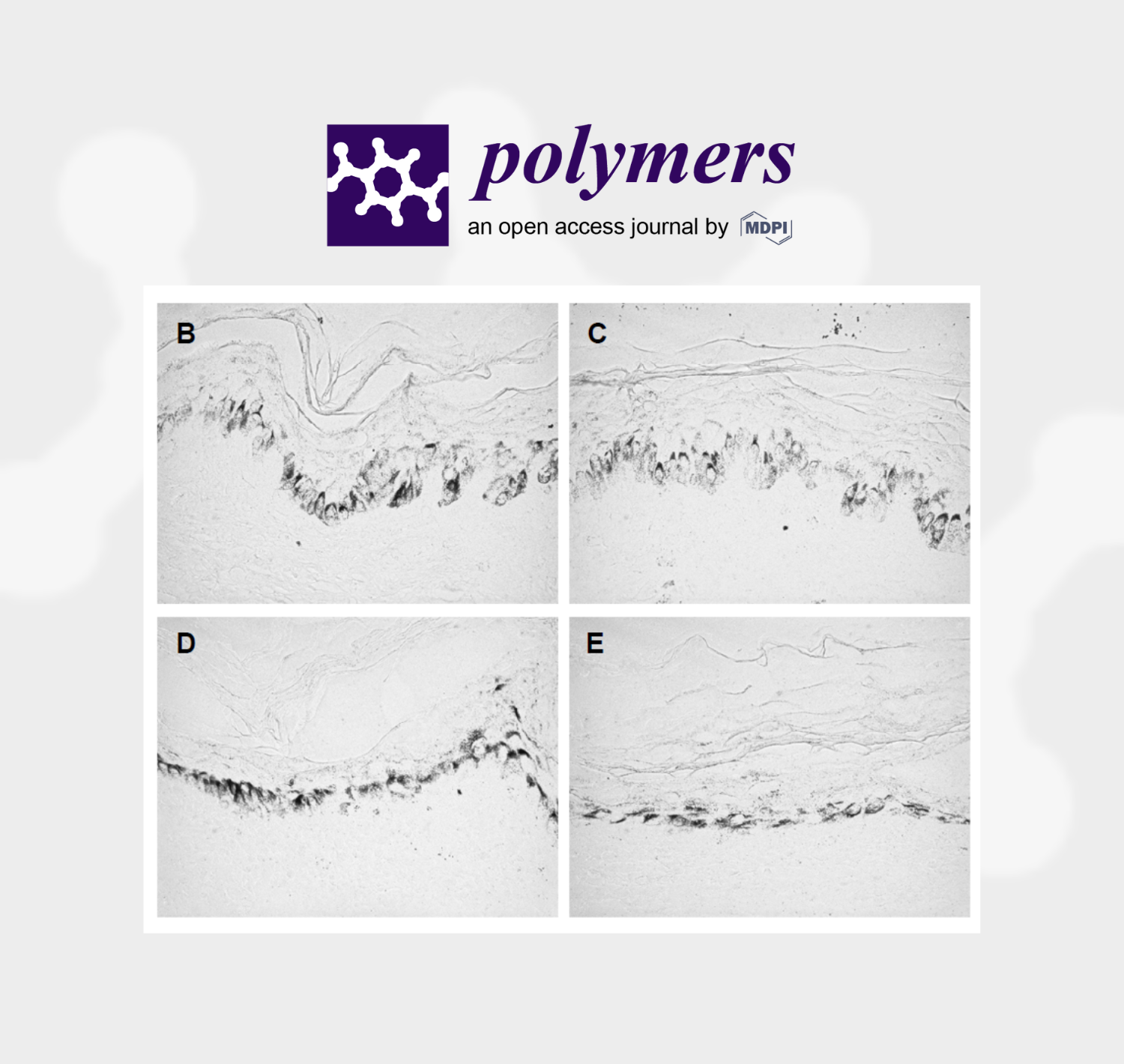
Issue 11: CELLBOOSTER® GLOW: Anti-Aging and Depigmentation Effect of a Hyaluronic Acid Mechanically Stabilized Complex on Human Skin Explants
Gabriel Siquier-Dameto 1,2,†, Sylvie Boisnic 3,†, Pere Boadas-Vaello 2 and Enrique Verdú 2,*
1 Dameto Clinics International, 1171 VC Badhoevedorp, The Netherlands; info@dametoclinics.com
2 Research Group of Clinical Anatomy, Embryology and Neuroscience (NEOMA), Department of Medical
Sciences, University of Girona, E-17003 Girona, Catalonia, Spain; pere.boadas@udg.edu
3 Groupe de Recherche et d’Evaluation en Dermatologie et Cosmétologie (GREDECO), 75116 Paris, France;
gredeco@orange.fr
* Correspondence: enric.verdu@udg.edu
† These authors contributed equally to this work.
Abstract: Solar radiation and environmental pollutants are factors that cause changes in the skin
that trigger skin aging. The objective of the study is to evaluate the rejuvenating effects of a complex
formed by hyaluronic acid supplemented with vitamins, amino acids and oligopeptides in explants
of human skin. For this, surplus skin samples have been obtained from donors that have been resected
and cultivated on slides with membrane inserts. The complex was administered to some skin
explants and the percentage of cells with low, medium and high levels of melanin was evaluated as
an indicator of the degree of pigmentation. Other skin segments were irradiated with UVA/UVB,
then the product was administered on several slides and the levels of collagen, elastin, sulfated GAG
and MMP1 were evaluated. The results show that the administration of the complex significantly
reduces the percentage of skin cells with a high melanin content by 16%, and that in skin irradiated
with UVA/UVB, there is a decrease in the content of collagen, elastin and sulfate GAGs, and the
complex reverses this reduction without changing MMP1 levels. This suggests that the compound
has anti-aging and depigmentation effects on the skin, giving a skin rejuvenation appearance.
Keywords: skin explants; melanin; extracellular matrix; hyaluronic acid; aging; depigmentation
————————-
1. Introduction
Hyaluronic acid (HA) is one of the most widely used biopolymers of extracellular
matrix components in dermo-aesthetic medicine, with the aim of rejuvenating the skin [1–
4]. In human skin, hyaluronic acid is located in the epidermis, except in the upper granular
layer and the stratum corneum, and in the first layers of the dermis (upper and lower
dermis) [5,6]. Hyaluronic acid content decreases with aging, both in men’s and women’s
skin [7], so its injection improves the aged appearance of the skin, especially to smooth
wrinkles. In this context, it has been observed that dermal injection of chemically crosslinked
hyaluronic acid (CL-HA) fills spaces, smooths wrinkles and favors collagen formation
by skin fibroblasts [8]. CL-HA injections revealed increased collagen formation
around the filler [9]. Injection of chemically cross-linked high-molecular-weight HA supplemented
with 0.3% lidocaine hydrochloride increases skin volume, improving the aesthetic
appearance of the skin [10]. Intradermal injections of cross-linked HA cause a reduction
in wrinkles [11]. Injections of non-cross-linked HA also improve skin hydration
and elasticity. In the periorbital area, they cause an improvement of between 25% and 50%
in skin brightness, texture and turgor [12,13]. The facial application of Viscoderm® Hydrobooster
(IBSA SA, Collina dʹOro, Switzerland), slightly cross-linked HA, induces a significant
improvement in facial wrinkles and static facial lines [14].
Non-animal stabilized hyaluronic acid (NASHA) injection improves skin texture [15]
and skin elasticity [16]. The subcutaneous injection of low-molecular-weight hyaluronic
acid fragments mixed with amino acid (HAAM) in aged skin improves the aesthetic appearance
of the skin, since it induces the production of collagen III by skin fibroblasts [17].
HA and hydroxyapatite complexes have also been used as skin fillers [18–22]. Combined
HA with glycerol improves skin quality by altering viscoelastic skin properties and skin
density [23], and HA plus mannitol also is effective for skin hydration [24].
Using CD1 mice, the effect of various HAs has been studied, such as Viscoderm 0.8 a
linear HA with a molecular weight of about 1 × 106 Da, Profhilo a hybrid cooperative complex-
based compound with low-molecular-weight HA (≈65–110 kDa) and high-molecular-
weight HA (≈1.4–2.1 × 106 Da), Profhilo Structura a hybrid cooperative complex-based
compound containing low- and high-molecular-weight HA and Aliaxin GP a cross-linked
HA (molecular weight 1000 kDa–2000 kDa). This study shows that the degradation kinetics
of subcutaneous implants of these four types of HA are 4, 10, 29 and greater than 33
weeks post-injection for Viscoderm, Profhilo, Profhilo Structura and Aliaxin GP, respectively.
This suggests that HA fillers with high molecular weight or a mixture of high and
low molecular weight have a greater capacity for skin integration [25]. Likewise, in guinea
pigs, the intradermal administration of Aliaxin GP, composed of cross-linked HA, and
Viscoderm® Skinkò E (SE), composed of non-cross-linked HA supplemented with ions,
amino acids and vitamins, shows that the injection of Aliaxin GP has slow reabsorption,
inducing scratching of the skin. In the skin of these animals treated with Aliaxin GP, no
increase in infiltrates was observed compared to the control group treated with saline solution.
In animals treated with Viscoderm® SE, reabsorption is rapid, with slight post-injection
erythema being observed. At the histological level, an increase in the deposit of
collagen, elastic fibers and proliferation of fibroblasts in the area of administration is observed,
with no increase in infiltrates compared to the control group [26].
All these studies are an example of the multiple types of HA used in aesthetic medicine
to rejuvenate the skin, ranging from non-cross-linked HA, cross-linked HA, autocross-
linked HA, as well as low- and high-molecular-weight HA, and supplemented HA
with amino acids, ions and vitamins, and preclinical studies provide valuable information
on the skin behavior of these HA-based compounds. In summary, this diversity of HAbased
products with different molecular weights and rheological characteristics constitutes
very useful tools in aesthetic medicine to reduce, delay and partially repair age-related
skin changes [27]. In the present study, the effect of treatment with CELLBOOSTER®
Glow (Suiselle SA, Yverdon-les-Bains, Switzerland), a revitalizing complex of hyaluronic
acid, made up of HA, amino acids (cysteine, glycine, lysine, proline and valine) oligopeptides
(glutathione) and vitamins (C, biotin), has been tested. CELLBOOSTER® Glow (CG)
is made up of high-molecular-weight hyaluronic acid, not cross-linked and mechanically
stabilized by shear deformation and simultaneous pressure. The objective of the study
was to demonstrate the efficacy of this revitalizing HA complex in skin depigmentation
and anti-aging when injected into the skin of human donors. The results show that in
human skin explants, treatment with CG significantly reduces the percentage of cells with
a high melanin content, which suggests a depigmentation effect, and that in explants irradiated
with UVA/UVB, treatment with CG increases the levels of components of the dermal
extracellular matrix, such as collagen type 1, elastin and sulfated GAGs, components
that had decreased in irradiated explants. Taken together, these results suggest that CG
has a depigmentation and rejuvenation and/or revitalization effect on the skin.
2. Materials and Methods
2.1. Human Skin Samples Collection and In Vitro Maintenance of Skin Explants
In the present study, skin samples from four female donors between 29 and 57 years
of age have been used. The human skin used is excess skin from abdominoplasty (skin #1;
50 years old), from tight lift (skin #2; 57 years old) and breast reduction (skin #3, 30 years old, and skin #4, 29 years old).
The donor women signed an informed consent in which they accepted that the resected skin from their interventions
could be used for scientific purposes. All procedures performed in this study were in accordance with the ethical
standards of the Helsinki Declaration.
In the first hour after excision of the skin, under a laminar flow hood and under sterile
conditions, the skin explants were washed with a PBS–antibiotic solution, and the subcutaneous
fat and lower dermis were mechanically removed using a surgical scarpel. Under
these conditions, the skin was cut into small fragments of approximately 1 cm2, which
were placed inside plate inserts with a 3 μm pore membrane (# 10769-210; VWR, Rosnysous-
Bois, France) in 12-well culture plates (Costar, VWR, France). The culture medium
was placed at the bottom of the wells, ensuring skin survival by slow diffusion between
the two compartments through the porous membrane. Medium changes were made 3
times per week. The culture medium used was Dulbecco’s Modified Minimal Essential
Medium (DMEM; Life Technologies, Saint-Aubin, France) enriched with antibiotics (penicillin
100 μg/mL, streptomycin 100 μg/mL and amphotericin B 250 μg/mL; Life Technologies),
bovine pituitary extract (Life Technologies), L-glutamine (200 μg/mL; Sigma-Aldrich-
Merck, Saint-Quentin-Fallavier, France) and fetal calf serum (Sigma-Aldrich-
Merck). The culture plates were placed in a humidified incubator in a 5% CO2 atmosphere
at 37 °C. In each experimental series, the control and test conditions were compared between
skin fragments from the same donors [28,29].
2.2. Experimental Series and Experimental Design
The effect of CELLBOOSTER® Glow has been tested in two experimental series of
human skin explants. In the first experimental series, the depigmentation effect has been
analyzed, for which 3 administrations of 10 μL per cm2 of the product were injected in the
superficial dermis and in the upper part of the middle dermis, of the skin samples. For
each donor, duplicates of skins treated with CG and control skins non-treated were made.
The day of the injection was considered as day 0 (D0), and the depigmentation effect was
evaluated during the following 12 days post-injection. Subsequently, skin samples were
formalin-fixed and histologically processed to detect melanin pigment.
In the second experimental series, the anti-aging effect of CG was studied, using a
model of aging by ultraviolet radiation. For this, the skin samples were irradiated with
ultraviolet A light (UVA; 8 J/cm2) and ultraviolet B light (UVB; 1 J/cm2) for 60 min by UVA
and 2 min by UVB. The source of ultraviolet irradiation was a Vilber Lourmat simulator
(Vilber Lourmat, Marnes-la-Vallée, France) fitted out with a UVA irradiation source (365
nm) composed of Vilber Lourmat tubes T-20.L-365 mercury vapor tubes, low pressure.
The UVB irradiation source (312 nm) is composed of Vilber Lourmat tubes T-20.L-312
mercury vapor tubes. A radiometer was associated with a microprocessor programmable
in energy (J/cm2). The skin samples have been irradiated with UVA and UVB because they
are the ultraviolet radiations that have the greatest penetrating capacity in the skin, ranging
from the epidermis to the dermis, and even the hypodermis. These ultraviolet radiations
generate free oxygen radicals, and this oxidative stress is responsible for skin aging
[30–32]. The day of radiation of the skin samples was considered day 0 (D0). After the
ultraviolet radiation session, the skin samples received 3 injections of 10 μL/cm2 in the
superficial dermis and in the upper part of the mid-dermis of CG. Irradiated non-treated
skin and non-irradiated skin also were used as controls. The anti-aging effect of the skin
has been studied during the 12 days following irradiation. Between days 1 and 4 (D1-D4),
the culture media were collected, mixed and frozen. These media were used for the analysis
of metalloproteinase type 1 (MMP1) activity. On day 4 (D4), skin fragments were frozen,
which were used for the analysis of glycosaminoglycans (GAGs). Finally, on day 12
(D12), the skin fragments were frozen and used for pro-collagen-I and elastin analysis.
2.3. Histological Evaluation of the Melanin Pigment of the Skin
Skin samples treated with CG and control skin samples were fixed in formalin and
subsequently embedded in paraffin. The paraffin blocks with the skin inside were cut to
a thickness of 4 μm and collected on pregelatinized slides. Histological sections of skin
were stained with the method of Fontana-Masson silver (FMS) stain. FMS stain is a histochemical
technique that oxidizes melanin and melanin-like pigments as it reduces silver
[33]. This histochemical method has been used to determine the degree of depigmentation
induced by CG. For this, the histological sections have been deparaffinized and rehydrated
with distilled water. Next, the Fontana-Masson stain kit (#HT200-1KT; Sigma-Aldrich-
Merck) was used, and the procedure described by the manufacturer was followed.
Finally, the histological sections were dehydrated in increasing solutions of ethanol,
bathed for 5 min in xylene, and coverslips were mounted with DPX (#06522; Sigma-Aldrich-
Merck).
A quantitative numeration of cells containing melanin pigments (black stain) was
made under an optical microscope at x400 magnification on about 300 basal cells of the
epidermis. Three types of cells were counted: unpigmented cells or cells presenting rare
melanin pigments isolated in the cytoplasm (score 1); cells presenting moderate melanin
pigment in all over the cytoplasm (score 2) and cells presenting important melanin pigment
in all over the cytoplasm (score 3). Then the epidermal melanin content was calculated
as the percentage of the epidermal cells in each cell type counted.
2.4. Biochemical Evaluation of Skin Samples
2.4.1. Biochemical Assay of Type 1 Pro-Collagen
During the aging process, the metabolism of fibroblasts is reduced. The objective of
an anti-aging product such as CG is to stimulate this metabolism in order to achieve collagen
and other extracellular matrix molecules synthesis. Thus, the analysis of the excretion
of the single-stranded molecule of type I alpha 1 pro-collagen, before the formation
of the triple helix, constitutes an interesting approach to evaluate an anti-aging effect. For
it, the skin fragments collected at D12 were weighed and then put in PBS (0.1 M, pH = 7.4)
After grinding with a potter, the amount of type I pro-collagen (μg/mL) was evaluated by
an ELISA method (# DY6220-05; Human Pro-Collagen I alpha 1, BioTechne), following the
procedure described by the manufacturer. The final result was expressed in pg of type 1
pro-collagen/mg of biopsy.
2.4.2. Biochemical Assay of Elastin
Elastin, the main component of elastic fibers, provides stretch and elasticity to the
skin. During the aging process, the synthesis of elastin decreases which impacts skin structure,
function and youthful appearance. The objective of an anti-aging product such as
CELLBOOSTER® Glow is also to stimulate the synthesis of elastin. Therefore, the assessment
of the elastin content in skin samples constitutes an interesting contribution to assess
the anti-aging effect.
After the 12-day survival period, insoluble elastin was extracted from skin samples
with 0.25 M oxalic acid at 100 °C as soluble alpha-elastin polypeptide fragments. After
centrifugation to remove undigested tissue, the Fastin Interchim Elastin Assay Kit (#F200;
Biocolor, Montluçon Cedex, France) was used, following the manufacturer’s procedure.
Finally, the amount of soluble elastin was measured by a spectrocolorimetric assay
method at 513 nm. To compare the different results, the quantity of elastin was related to
the quantity of total proteins in the sample. The protein concentration (μg/mL) was determined
spectrophotometrically at 562 nm, using the Pierce BCA protein assay kit (# 23225;
ThermoFisher, Waltham, MA, USA), and following the procedure indicated by the manufacturer.
Finally, results were expressed in μg elastin/mg protein.
2.4.3. Biochemical Assay of Sulfated Glycosaminoglycans
In this assay, frozen skin fragments from day 4 (D4) have been used. These fragments
were enzymatically digested with papain at 50 °C, overnight. And then, the activity of the
fibroblasts to synthesize sulfated GAGs was evaluated by a spectrocolorimetric method at
656 nm, using the Sircoll blyscan GAGs kit (#AA4881; Bicolor, Montluçon Cedex, France),
and following the procedure indicated by the manufacturer. To compare the different results,
the amount of GAGs was related to the amount of total protein in the sample. The
assay of the protein concentration was carried out spectrophotometrically at 562 nm, using
the Pierce BCA protein assay kit (# 23225; ThermoFisher, Waltham, MA, USA), and
following the procedure indicated by the manufacturer. The results were expressed in μg
of sulfated GAGs/mg protein.
2.4.4. Biochemical Assay for the Analysis of Metalloproteinase Type 1 (MMP1) Activity
Metalloproteinases are enzymes involved in the degradation of macromolecules of
the extracellular matrix. The interstitial collagenase of fibroblasts MMP1 is specific to type
I, II and III fibrillar collagens. MMP1 is first secreted into the culture medium by the fibroblast
in its zymogenic form, pro-MMP1, which is then activated into MMP1 by the action
of other proteinases.
In this assay, the culture media collected between days 1 and 4 (D1-D4) have been
used. This culture medium has been thawed and centrifuged at 900 rpm to remove potential
cell debris and other impurities. The amount of MMP1 (pg/mL) contained in the supernatant
has then been analyzed by the ELISA technique with a spectrophotometric
reader at 450 nm, and using the Human MMP-1 (Matrix Metalloproteinase 1) ELISA Kit
(# E-EL-H6073; Elabscience Biotechnology Inc, Houston, TX, USA), and following the procedure
described by the manufacturer. The final result was expressed in pg of MMP1/mg
of biopsy.
2.5. Statistical Analysis
The mean and standard deviation (SD) were calculated in tests performed in doublet
in 4 donors in duplicate (8 values). After checking the normality of the groups using the
Shapiro–Wilk test, the comparison of the parameter was made with the Student’s T test
(alpha risk of 5%). If the groups did not follow a normal distribution, a Wilcoxon test (5%
alpha risk) was performed. The IBM SPSS 25.0 statistical package for Windows (IBM Corp.
Released 2017; Armonk, NY, USA) was used for the statistical analysis.
3. Results
3.1. Treatment with CELLBOOSTER® Glow Significantly Reduces the Percentage of Skin Cells
with High Melanin Content
In human skin explants, three injections of CG were administered. The percentage of
cells with a melanin score of 1 was 18.30% and 21.93% in the control and treated groups,
respectively. No significant differences were observed between both groups (p > 0.05). Regarding
the percentage of pigmented cells with a score of 2, it was 42.83% and 45.52% in
the control and treated groups, respectively. Neither were significant differences observed
between both experimental groups (p > 0.05). Finally, the percentage of cells with a score
of 3 was 38.87% and 32.55% in the control and treated groups, respectively. For this score,
significant differences were observed between both experimental groups (p < 0.05) (Figure
1). CG treatment does not reduce the percentage of skin cells with low and medium melanin
content (scores 1 and 2); however, it does significantly reduce the percentage of skin
cells with significant melanin content (score 3). This reduction is approximately 16%.
Taken together, these results indicate that CG treatment slightly but significantly reduces
skin pigmentation. This indication may favor a less-aged cosmetic appearance.
Figure 1. Histological results of skin pigmentation: (A) Histogram of the percentage of cells with
low (score 1), medium (score 2) and high (score 3) melanin content in both experimental groups
(Control, CBG). Values are mean ± standard deviation (n = 8 values). * p < 0.05 compared to the
control group. (B,C) Light microscope images of skin donor #1, and (D,E) images of skin donor #2.
Images (B,D) correspond to the control, and images (C,E) after CG treatment. Comparatively, a
slight decrease in black marking (cells with melanin) can be observed in images (C,E) compared to
images (B,D). All images were captured at ×400 magnification.
3.2. In Skin Treated with Ultraviolet Radiation, Treatment with CELLBOOSTER Glow Significantly
Increases the Content of Pro-Collagen Type I, Elastin and Sulphated GAGs, but without
Causing Changes in MMP1 Levels
Ultraviolet irradiation causes a significant decrease in the levels of type I pro-collagen,
elastin and sulfate GAGs in human skin explants compared to the control, which
corresponds to non-irradiated skin (Figure 2A–C), while it significantly increases the levels
of MMP1 compared to the control (Figure 2D).
Figure 2. Biochemical results of skin irradiated with UVA/UVB and treated with CELLBOOSTER®
Glow (CG): (A) Histogram of the results of the content of pro-collagen type I in control, irradiated
with ultraviolet, and in skin irradiated with ultraviolet and treated with CG; (B) histogram of the
elastin content and (C) of sulfated GAGs, in the skin in the same three experimental groups; (D)
histogram of MMP1 activity and/or content in the skin of the three experimental groups. * p < 0.05;
** p < 0.01; *** p < 0.001. Values are mean ± standard deviation (n = 8 values).
When UV-irradiated skin is treated with CG, the levels of pro-collagen type 1, elastin
and sulfated GAGs increase compared to irradiated skin without treatment, to levels similar
to those observed in the control group (Figure 2A–C). However, MMP1 levels remained
similar between the groups of skin irradiated with and without CG treatment
(Figure 2D). Ultraviolet irradiation of the skin caused a reduction of 28.9%, 13.2% and
19.2% in the content, respectively, pro-collagen type 1, elastin and GAGs, compared to
what was observed in the control group. Likewise, in ultraviolet-irradiated skin, treatment
with CG caused a 47.9%, 25.3% and 22.4% increase in the content of pro-collagen type 1,
elastin and GAGs, respectively, compared to what was observed in untreated irradiated
skin (UV group). In skin irradiated with ultraviolet, a significant increase of 40.9% in the
level of MMP1 was observed, compared to what was observed in the control, while in the
UV + CBG group, a non-significant reduction of 6.7% was observed compared to the UV
group. Taken together, these biochemical results suggest that ultraviolet irradiation of the
skin causes changes compatible with aging that manifest as a reduction in the content of
pro-collagen type 1, elastin and sulfated GAGs, as well as an increase in MMP1 activity,
and the CG treatment reverses these changes by increasing the levels of these extracellular
matrix components, except for MMP1 activity, which can be attributed to its rejuvenating
effect. In summary, treatment with CG in skin irradiated with ultraviolet radiation increases
the levels of pro-collagen type 1, elastin and sulfate GAGs, without modifying the
activity of MMP1.
4. Discussion
In the present study, it has been observed that in human skin explants, treatment
with CG significantly reduces skin pigmentation, especially a significant reduction of 16%
of cells with high melanin content has been observed. And in skin treated with ultraviolet
A and B radiation, CG treatment significantly increases the content of components of the
extracellular matrix of the dermis–epidermis, especially collagen type 1, elastin and sulfated
GAGs. All these changes observed in the skin after treatment with CG suggest that
this treatment promotes skin rejuvenation.
CELLBOOSTER® Glow (CG) consists of non-cross-linked and mechanically stabilized
HA supplemented with amino acids and vitamins, specifically cysteine, glycine, lysine,
proline and valine amino acids, glutathione as an oligopeptide, and as vitamins it
contains biotin and vitamin C. The observed effects in the present study may be due to
the components of CG. In this context, it is known that ultraviolet radiation causes an
increase in the levels of enzymes that degrade the extracellular matrix of the skin, such as
collagenases, gelatinases and metalloproteinase type I, which leads to a decrease in collagen
content and unstructured resynthesis of this collagen [34]. Ultraviolet radiation also
causes a reduction in the release of elastase by skin fibroblasts, so the structural changes
that this radiation induces on the elastic fibers of the skin are not easily degraded, which
become curly and tortuous, losing skin elasticity and maintaining wrinkles [35]. Ultraviolet
radiation triggers the aggregation of elastin. Elastin aggregates are not eliminated by
the set of extracellular chaperones present in the skin, because ultraviolet radiation also
induces the inactivation of these chaperones. Consequently, these elastin aggregates remain
in the skin and this decreases its elasticity [36]. Taken together, all these findings
suggest that the skin’s elasticity is compromised by ultraviolet radiation from the sun,
giving it a more aged appearance, with the formation of wrinkles. Likewise, ultraviolet
radiation also induces a reduction in the expression of hyaluronic synthase enzymes (type
1, 2 and 3), transforming growth factor beta-1, and its type II receptor. All this leads to a
lower proliferation of fibroblasts, with a lower synthesis and release of components of the
extracellular matrix (e.g., HA and collagen) [37–39]. Finally, an in vitro study demonstrated
that ultraviolet radiation also causes a reduction in elastin content [40] and a
reduction of GAGs in cultured human fibroblasts [41]. In the present study, it has been
observed that in human skin explants ultraviolet radiation reduces the content of elastin,
type I collagen and sulfated GAGs. In turn, it is known that sulfated GAGs play an important
role in establishing interactions with other components of the extracellular matrix,
allowing them to retain water, thus maintaining the degree of dermal hydration; retain
growth factors and act as co-receptors of these growth factors, responsible for the survival
of skin cells; and modulate the inflammatory–immune response of the skin by interacting
with cytokines–chemokines [42]. Consequently, the reduction of these sulfated GAGs in
skin samples subjected to ultraviolet radiation can compromise all these functions, favoring
the appearance of skin signs associated with aging.
As in the present study, other previous studies have also observed an increase in
MMP1 levels in UV-irradiated skin samples [43,44] and in cultured human fibroblasts [45].
Matrix metalloproteinase type 1 (MMP1) belongs to the collagenase subgroup and its
function is to degrade type I and type III collagen [46]. Type I collagen is synthesized and
secreted by dermal fibroblasts and represents 80–90% of skin collagen. Fibroblasts also
synthesize and release type III collagen, which represents 15% of the collagen in the skin.
Both are of the fibril-forming types. Collagen fibers form extensive and robust networks
providing the dermis with strength, firmness and elasticity. A collagen fiber is essentially
composed of bundles of smaller fibrils. Collagen fibrils are approximately 10 to 300 nm in
diameter and several micrometers in length. A collagen fibril is a bundle of triple-stranded
collagen molecules (about 1.5 nm in diameter and approximately 300 nm long). This triple-
helix, coiled structure is stereo-dynamically favorable to allow strands to be interwoven
together and this incredibly robust structure can persist in tissues for many years. The
formation of fibers is dependent on interaction with other ECM components including
elastin proteins and GAGs [47]. The increase in MMP1 after ultraviolet irradiation of the
skin suggests a degradation of these collagen fibers, a loss of mechanical firmness of the
skin and the ability to stretch the skin, as well as a decrease in skin elasticity, and all these
cause the appearance of signs of skin aging.
Treatment with CG reverses the observed effects of ultraviolet radiation to levels
comparable to control. Specifically, the levels of type I collagen, elastin and sulfated GAGs
are restored, although it does not cause a decrease in MMP1. The increased levels of biopolymers
in the ECM can be explained by a reactivation of skin fibroblasts by the CG
components. In vitro studies show that HA promotes the proliferation of fibroblasts [48–
52] that secrete a greater amount of type I collagen [52]. HA binds to the CD44 receptor,
expressed by dermal fibroblasts [53], and attenuates MMP1 overexpression [54]. HA can
also bind the CD44 receptor on keratinocytes [55] inducing the growth, survival and migration
of these epidermis cells [56], which implies an acceleration of the epidermal rejuvenation
process. Likewise, the interaction of HA with the CD44 receptor of the keratinocyte
also favors the formation and release of lamellar bodies into the extracellular space
of the epidermis, which favors the maintenance of the degree of hydration and the rejuvenation
of the epidermis [57]. Epidermal melanocytes also express CD44 receptors [58],
and sulfate GAGs enhance the melanotic phenotype of these cells through binding to
CD44 receptors [59]. In a physiological situation, melanocytes have a layer of hyaluronic
acid that surrounds them and that prevents them from synthesizing and secreting cytokines
and chemokines. Ultraviolet radiation favors hyaluronidases to degrade HA and
melanocytes to secrete these pro-inflammatory factors [60]. It is well known that pro-inflammatory
factors, especially cytokines, play a crucial role in the manifestation of decreased
skin collagen content, decreased skin thickness and dry and wrinkled skin [61].
Therefore, the application of CG, which provides hyaluronic acid, can potentially restore
this HA layer in the melanocytes, preventing these epidermal cells from secreting the inflammatory
cytokines that trigger the signs of skin aging. Preclinical evidence indicates
that the suppression of the synthesis and release of pro-inflammatory cytokines by melanocytes
would be mediated by the binding of HA to CD44 receptors [60]. In addition, in
vitro studies show that HA prevents the secretion of inflammatory cytokines by
Polymers 2023, 15, 2438 9 of 13 keratinocytes induced by ultraviolet radiation [62] and ionizing radiation [63].
Taken together, all these evidences suggest that the HA biopolymer present in CG has a skin rejuvenation
effect through its interaction with CD44 receptors, favoring the proliferation of
dermal fibroblasts and the release of extracellular matrix proteins (type I and III collagen,
sulfated GAGs, elastin) and preventing the release of inflammatory cytokines by epidermal
melanocytes and keratinocytes.
CELLBOOSTER® Glow also contains biotin and vitamin C. Biotin promotes protein
biosynthesis in the liver, intestinal wall and skin [64]. Fibroblasts require biotin for their
survival and proliferation [65]. In animals subjected to a diet deficient in biotin, alterations
in the composition of fatty acids of the skin are observed, such as accumulation of oddchain
fatty acids and abnormal metabolism of long-chain polyunsaturated fatty acids
[66,67]. In these animals, a loss of Langerhans cells in the epidermis is also observed,
which compromises the innate immune system of the skin [68]. On the other hand, vitamin
C plays different biological functions in the skin, such as promoting collagen synthesis
and structural stabilization of collagen fibers; it is a powerful antioxidant that neutralizes
and removes oxidant molecules; reduces the synthesis of melanin in melanocytes and
facilitates the proliferation and differentiation of keratinocytes and the release of lipids
that surround the corneocytes from the stratum corneum of the epidermis, thus facilitating
the renewal of the epidermis layers [69]. Vitamin C inhibits the hyaluronidase enzymes
responsible for the breakdown of hyaluronic acid in the epidermis [70]. Furthermore,
skin pigmentation requires multiple steps, namely, the activation of melanocytes,
the synthesis of melanin, the transport of melanosomes to the tips of melanocyte dendrites
and the transfer of melanosomes from melanocytes to surrounding keratinocytes. It has
been shown that vitamin C inhibits melanin synthesis through downregulation of tyrosinase
enzyme activity [71]. Vitamin C also interferes with the transport of melanosomes to
the tips of the dendrites of melanocytes, since this vitamin reduces the expression of
transport proteins of these melanosomes (e.g., kinesin) [72]. All these findings suggest that
these two vitamins present in CG promote the activation of dermal fibroblasts and with it
the synthesis of new extracellular matrix proteins; they maintain the proliferation of
keratinocytes allowing adequate renewal of the epidermis, as well as the lipid content of
the stratum corneum of the epidermis, which contributes both to epidermal renewal and
to maintaining the degree of skin hydration; they prevent the degradation of the HA that
surrounds the melanocytes, preventing the release of inflammatory cytokines and they
decrease the synthesis of melanin and the transport of melanosomes to the dendritic ends
of the melanocytes, which has a depigmentation effect. All of these changes can contribute
to a more rejuvenated appearance of the skin. Regarding skin pigmentation, in the present
study, it has been verified that CG reduces the percentage of epidermal cells with a high
melanin content and limits the percentage of cells with a low and intermediate content.
Cysteine, glycine, lysine, proline and valine are the amino acids present in CG. Cysteine
is an important amino acid in the synthesis of keratins by the keratinocytes of the
epidermis. Lysine is an amino acid that is incorporated into skin proteins and post-translational
changes of this residue allow the maturation of these skin proteins. The oxidation
of lysine allows cross-linking of the collagen fibers, which gives the stretching strength
and insolubility of these protein fibers. Glycine and proline are the two most abundant
amino acids in collagen fibers. Proline also undergoes post-translational changes allowing
the maturation of collagen fibers. Proline is also an amino acid that promotes skin elasticity
[73]. Additionally, glutathione that is present in CG is a tripeptide made up of the
amino acid glutamate, cysteine and glycine. It has been shown that glutathione (GSH) not
only acts as an antioxidant by scavenging free radicals, but it is also involved in
pheomelanin formation and regulating melanogenesis [74]. Oral administration of GSH
resulted in the lightening of skin color in humans [75]. Moreover, the oxidized form of
glutathione (GSSG) was also found to have anti-melanogenic effects in humans [76,77].
All these evidences suggest that GSH present in CG has anti-aging effects on the skin,
sequestering free radicals that cause cell damage that accelerate skin aging, as well as
reducing the degree of skin pigmentation by having anti-melanogenic effects, all these
contributing to skin rejuvenation. GSH may also contribute to the depigmentation results
observed in the present study.
The evidence described above suggests that CG components have skin rejuvenating
effects, at least on human skin explants. A good part of the changes observed may be due
to the effect of hyaluronic acid on the different cellular elements of skin explants and, to a
lesser extent, to vitamins, amino acids and oligopeptides. Recently, newer technologies in
transdermal delivery systems had become effective [78] for skin rejuvenation. Nevertheless,
clinical studies are necessary to corroborate these effects of CG.
5. Conclusions
The objective of this study is to evaluate the rejuvenating effects of a stabilized compound
formed by HA supplemented with vitamins, amino acids and oligopeptide in human
skin explants. Transformations in the skin, which activate skin aging, can be caused
by elements such as the sun’s ultraviolet light and atmospheric contaminants. HA is one
of the most widely used biopolymers of extracellular matrix components in dermo-aesthetic
medicine, with the aim of rejuvenating the skin. The effect of various HAs has been
studied in vivo and in vitro. In the present study, the effect of treatment with CELLBOOSTER
® Glow (CG) has been tested when injected into the skin of human donors. CG
is made up of high-molecular-weight hyaluronic acid, non-cross-linked and mechanically
stabilized. The results show that in human skin explants, treatment with CG significantly
reduces the percentage of cells with a high melanin content, which confirms a depigmentation
effect of the formula. In explants irradiated with UVA/UVB, treatment with CG increases
the levels of components of the dermal extracellular matrix, such as collagen type
1, elastin and sulfated GAGs, components that had decreased in irradiated explants. Taken
together, these outcomes suggest that CG has a depigmentation and rejuvenation and/or
revitalization effect on the skin. This indication of a slight reduction of skin pigmentation
and an increase of ECM components may favor a less-aged cosmetic appearance.
Author Contributions: All authors have contributed sufficiently to be included as authors. Conceptualization,
methodology and validation, S.B. and G.S.-D.; formal analysis and investigation, G.S.-
D., S.B., P.B.-V. and E.V.; resources, S.B. and E.V.; data curation, G.S.-D., S.B., P.B.-V. and E.V.; writing—
original draft preparation, E.V. and P.B.-V.; writing—review and editing, G.S.-D., S.B., P.B.-V.
and E.V.; visualization, G.S.-D., P.B.-V. and E.V.; supervision, G.S.-D., S.B., P.B.-V. and E.V.; project
administration, S.B. and E.V.; funding acquisition, G.S.-D., S.B., E.V. and P.B.-V. All authors have
read and agreed to the published version of the manuscript.
Funding: This research was funded by Suiselle SA, Rue Galilée 6, 1400 Yverdon-les-Bains, Switzerland
(grant number 88.7.22 and grant number 045/22).
Institutional Review Board Statement: The study was conducted in accordance with the Declaration
of Helsinki. The patients who participated in this study signed an informed consent in which
they gave up the excess skin from the operations they underwent for scientific studies. As the present
study has been carried out with these human skin explants, the experimental procedure has
not been required to be approved by an Ethics Committee.
Informed Consent Statement: Informed consent was obtained from all subjects involved in the
study.
Data Availability Statement: All data generated or analyzed during this study are included in this
published article.
Acknowledgments: The authors thank the entire GREDECO technical team (France) for technical
assistance in the realization of the present study.
Conflicts of Interest: The authors declare no conflict of interest.
References
1. Salwowska, N.M.; Bebenek, K.A.; Żądło, D.A.; Wcisło-Dziadecka, D.L. Physiochemical properties and application of hyaluronic
acid: A systematic review. J. Cosmet. Dermatol. 2016, 15, 520–526.
2. Bukhari, S.N.A.; Roswandi, N.L.; Waqas, M.; Habib, H.; Hussain, F.; Khan, S.; Sohail, M.; Ramli, N.A.; Thu, H.E.; Hussain, Z.
Hyaluronic acid, a promising skin rejuvenating biomedicine: A review of recent updates and pre-clinical and clinical investigations
on cosmetic and nutricosmetic effects. Int. J. Biol. Macromol. 2018, 120 (Pt B), 1682–1695.
3. Juncan, A.M.; Moisă, D.G.; Santini, A.; Morgovan, C.; Rus, L.L.; Vonica-Țincu, A.L.; Loghin, F. Advantages of Hyaluronic Acid
and Its Combination with Other Bioactive Ingredients in Cosmeceuticals. Molecules 2021, 26, 4429.
4. Bravo, B.; Correia, P.; Gonçalves Junior, J.E.; Sant’Anna, B.; Kerob, D. Benefits of topical hyaluronic acid for skin quality and
signs of skin aging: From literature review to clinical evidence. Dermatol. Ther. 2022, 35, e15903.
5. Juhlin, L. Hyaluronan in skin. J. Intern. Med. 1997, 242, 61–66.
6. Legouffe, R.; Jeanneton, O.; Gaudin, M.; Tomezyk, A.; Gerstenberg, A.; Dumas, M.; Heusèle, C.; Bonnel, D.; Stauber, J.; Schnebert,
S. Hyaluronic acid detection and relative quantification by mass spectrometry imaging in human skin tissues. Anal. Bioanal.
Chem. 2022, 414, 5781–5791.
7. Oh, J.H.; Kim, Y.K.; Jung, J.Y.; Shin, J.E.; Chung, J.H. Changes in glycosaminoglycans and related proteoglycans in intrinsically
aged human skin in vivo. Exp. Dermatol. 2011, 20, 454–456.
8. Cui, Y.; Wang, F.; Voorhees, J.J.; Fisher, G.J. Rejuvenation of Aged Human Skin by Injection of Cross-linked Hyaluronic Acid.
Plast. Reconstr. Surg. 2021, 147, 43S–49S.
9. Wang, F.; Garza, L.A.; Kang, S.; Varani, J.; Orringer, J.S.; Fisher, G.J.; Voorhees, J.J. In vivo stimulation of de novo collagen
production caused by cross-linked hyaluronic acid dermal filler injections in photodamaged human skin. Arch. Dermatol. 2007,
143, 155–163.
10. Sundaram, H.; Shamban, A.; Schlessinger, J.; Kaufman-Janette, J.; Joseph, J.H.; Lupin, M.; Draelos, Z.; Carey, W.; Smith, S.; Eaton,
L. Efficacy and Safety of a New Resilient Hyaluronic Acid Filler in the Correction of Moderate-to-Severe Dynamic Perioral
Rhytides: A 52-Week Prospective, Multicenter, Controlled, Randomized, Evaluator-Blinded Study. Dermatol. Surg. 2022, 48, 87–
93.
11. Alessandrini, A.; Tretyakova, K. The Rejuvenating Effect and Tolerability of an Auto-Cross-Linked Hyaluronic Acid on Décolletage:
A Pilot Prospective Study. Aesthetic Plast. Surg. 2018, 42, 520–529.
12. Ayatollahi, A.; Firooz, A.; Samadi, A. Evaluation of safety and efficacy of booster injections of hyaluronic acid in improving the
facial skin quality. J. Cosmet. Dermatol. 2020, 19, 2267–2272.
13. Succi, I.B.; da Silva, R.T.; Orofino-Costa, R. Rejuvenation of periorbital area: Treatment with an injectable nonanimal non-crosslinked
glycerol added hyaluronic acid preparation. Dermatol. Surg. 2012, 38, 192–198.
14. Sparavigna, A.; Tenconi, B.; Giori, A.M.; Bellia, G.; La Penna, L. Evaluation of the efficacy of a new hyaluronic acid gel on dynamic
and static wrinkles in volunteers with moderate aging/photoaging. Clin. Cosmet. Investig. Dermatol. 2019, 12, 81–90.
15. Belmontesi, M.; De Angelis, F.; Di Gregorio, C.; Iozzo, I.; Romagnoli, M.; Salti, G.; Clementoni, M.T. Injectable Non-Animal
Stabilized Hyaluronic Acid as a Skin Quality Booster: An Expert Panel Consensus. J. Drugs Dermatol. 2018, 17, 83–88.
16. Reuther, T.; Bayrhammer, J.; Kerscher, M. Effects of a three-session skin rejuvenation treatment using stabilized hyaluronic acidbased
gel of non-animal origin on skin elasticity: A pilot study. Arch. Dermatol. Res. 2010, 302, 37–45.
17. Scarano, A.; Sbarbati, A.; Amore, R.; Iorio, E.L.; Ferraro, G.; Marchetti, M.; Amuso, D. The role of hyaluronic acid and amino
acid against the aging of the human skin: A clinical and histological study. J. Cosmet. Dermatol. 2021, 20, 2296–2304.
18. Yutskovskaya, Y.; Kogan, E.; Leshunov, E. A randomized, split-face, histomorphologic study comparing a volumetric calcium
hydroxylapatite and a hyaluronic acid-based dermal filler. J. Drugs Dermatol. 2014, 13, 1047–1052.
19. Jeong, S.H.; Fan, Y.F.; Baek, J.U.; Song, J.; Choi, T.H.; Kim, S.W.; Kim, H.E. Long-lasting and bioactive hyaluronic acid-hydroxyapatite
composite hydrogels for injectable dermal fillers: Physical properties and in vivo durability. J. Biomater. Appl. 2016, 31,
464–474.
20. Chang, J.W.; Koo, W.Y.; Kim, E.K.; Lee, S.W.; Lee, J.H. Facial Rejuvenation Using a Mixture of Calcium Hydroxylapatite Filler
and Hyaluronic Acid Filler. J. Craniofac Surg. 2020, 31, e18–e21.
21. Felix Bravo, B.; Bezerra de Menezes Penedo, L.; de Melo Carvalho, R.; Amante Miot, H.; Calomeni Elias, M. Improvement of
Facial Skin Laxity by a Combined Technique with Hyaluronic Acid and Calcium Hydroxylapatite Fillers: A Clinical and Ultrasonography
Analysis. J. Drugs Dermatol. 2022, 21, 102–106.
22. Fakih-Gomez, N.; Kadouch, J. Combining Calcium Hydroxylapatite and Hyaluronic Acid Fillers for Aesthetic Indications: Efficacy
of an Innovative Hybrid Filler. Aesthetic Plast. Surg. 2022, 46, 373–381.
23. Kleine-Börger, L.; Hofmann, M.; Kerscher, M. Microinjections with hyaluronic acid in combination with glycerol: How do they
influence biophysical viscoelastic skin properties? Skin Res. Technol. 2022, 28, 633–642.
24. Taieb, M.; Gay, C.; Sebban, S.; Secnazi, P. Hyaluronic acid plus mannitol treatment for improved skin hydration and elasticity.
J. Cosmet. Dermatol. 2012, 11, 87–92.
25. Scrima, M.; Merola, F.; Vito, N.; Pacchioni, D.; Vecchi, G.; Melito, C.; Iorio, A.; Giori, A.M.; Ferravante, A. Elucidations on the
performance and reversibility of treatment with hyaluronic acid based dermal fillers: In vivo and in vitro approaches. Clin.
Cosmet. Investig. Dermatol. 2022, 15, 2629–2640.
26. Iannitti, T.; Morales-Medina, J.C.; Coacci, A.; Palmieri, B. Experimental and clinical efficacy of two hyaluronic acid-based compounds
of different cross-linkage and composition in the rejuvenation of the skin. Pharm. Res. 2016, 33, 2879–2890.
Polymers 2023, 15, 2438 12 of 13
27. Romagnoli, M.; Piersini, P.; Romano, D.; Bellia, G.; Siquier-Dameto, G. A line of different hyaluronans in skin chrono and photoaging:
A review of the literature and usage protocols. Aesthetic Med. 2022, 8, 36–43.
28. Boisnic, S.; Branchet-Gumila, M.C.; Benslma, L.; Le Charpentier, Y.; Arnaud-Battandier, J. Long term culture of normal skin to
test the efficacy of a hydroxy-acid-containing cream. Eur. J. Dermatol. 1997, 7, 271–273.
29. Boisnic, S.; Branchet, M.C.; Gouhier-Kodas, C.; Verriere, F.; Jabbour, V. Anti-inflammatory and antiradical effects of a 2% diosmin
cream in a human skin organ culture as model. J. Cosmet. Dermatol. 2018, 17, 848–854.
30. D’Orazio, J.; Jarrett, S.; Amaro-Ortiz, A.; Scott, T. UV radiation and the skin. Int. J. Mol. Sci. 2013, 14, 12222–12248.
31. Gromkowska-Kępka, K.J.; Puścion-Jakubik, A.; Markiewicz-Żukowska, R.; Socha, K. The impact of ultraviolet radiation on skin
photoaging–review of in vitro studies. J. Cosmet. Dermatol. 2021, 20, 3427–3431.
32. Salminen, A.; Kaarniranta, K.; Kauppinen, A. Photoaging: UV radiation-induced inflammation and immunosuppression accelerate
the aging process in the skin. Inflamm. Res. 2022, 71, 817–831.
33. Kwon-Chung, K.J.; Hill, W.B.; Bennett, J.E. New, special stain for histopathological diagnosis of cryptococcosis. J. Clin. Microbiol.
1981, 13, 383–387.
34. Fisher, G.J.; Wang, Z.Q.; Datta, S.C.; Varani, J.; Kang, S.; Voorhees, J.J. Pathophysiology of premature skin aging induced by
ultraviolet light. N. Engl. J. Med. 1997, 337, 1419–1428.
35. Imokawa, G. Recent advances in characterizing biological mechanisms underlying UV-induced wrinkles: A pivotal role of fibrobrast-
derived elastase. Arch. Dermatol. Res. 2008, 300 (Suppl. S1), S7–S20.
36. Janig, E.; Haslbeck, M.; Aigelsreiter, A.; Braun, N.; Unterthor, D.; Wolf, P.; Khaskhely, N.M.; Buchner, J.; Denk, H.; Zatloukal,
K. Clusterin associates with altered elastic fibers in human photoaged skin and prevents elastin from ultraviolet-induced aggregation
in vitro. Am. J. Pathol. 2007, 171, 1474–1482.
37. Dai, G.; Freudenberger, T.; Zipper, P.; Melchior, A.; Grether-Beck, S.; Rabausch, B.; de Groot, J.; Twarock, S.; Hanenberg, H.;
Homey, B.; et al. Chronic ultraviolet B irradiation causes loss of hyaluronic acid from mouse dermis because of down-regulation
of hyaluronic acid synthases. Am. J. Pathol. 2007, 171, 1451–1461.
38. Chen, M.; Zhang, G.; Yi, M.; Chen, X.; Li, J.; Xie, H.; Chen, X. Effect of UVA irradiation on proliferation and NO/iNOS system of
human skin fibroblast. Zhong Nan Da Xue Xue Bao Yi Xue Ban 2009, 34, 705–711.
39. Ju, M.; Chen, K.; Chang, B.; Gu, H. UVA1 irradiation inhibits fibroblast proliferation and alleviates pathological changes of
scleroderma in a mouse model. J. Biomed. Res. 2012, 26, 135–142.
40. Seite, S.; Zucchi, H.; Septier, D.; Igondjo-Tchen, S.; Senni, K.; Godeau, G. Elastin changes during chronological and photo-ageing:
The important role of lysozyme. J. Eur. Acad. Dermatol. Venereol. 2006, 20, 980–987.
41. Shin, J.E.; Oh, J.H.; Kim, Y.K.; Jung, J.Y.; Chung, J.H. Transcriptional regulation of proteoglycans and glycosaminoglycan chainsynthesizing
glycosyltransferases by UV irradiation in cultured human dermal fibroblasts. J. Korean Med. Sci. 2011, 26, 417–724.
42. Wang, S.T.; Neo, B.H.; Betts, R.J. Glycosaminoglycans: Sweet as Sugar Targets for Topical Skin Anti-Aging. Clin. Cosmet. Investig.
Dermatol. 2021, 14, 1227–1246.
43. Brennan, M.; Bhatti, H.; Nerusu, K.C.; Bhagavathula, N.; Kang, S.; Fisher, G.J.; Varani, J.; Voorhees, J.J. Matrix metalloproteinase-
1 is the major collagenolytic enzyme responsible for collagen damage in UV-irradiated human skin. Photochem. Photobiol. 2003,
78, 43–48.
44. Kim, D.J.; Iwasaki, A.; Chien, A.L.; Kang, S. UVB-mediated DNA damage induces matrix metalloproteinases to promote photoaging
in an AhR- and SP1-dependent manner. JCI Insight 2022, 7, e156344.
45. Song, X.Z.; Xia, J.P.; Bi, Z.G. Effects of (-)-epigallocatechin-3-gallate on expression of matrix metalloproteinase-1 and tissue inhibitor
of metalloproteinase-1 in fibroblasts irradiated with ultraviolet A. Chin. Med. J. 2004, 117, 1838–1841.
46. Pittayapruek, P.; Meephansan, J.; Prapapan, O.; Komine, M.; Ohtsuki, M. Role of Matrix Metalloproteinases in Photoaging and
Photocarcinogenesis. Int. J. Mol. Sci. 2016, 17, 868.
47. Reilly, D.M.; Lozano, J. Skin collagen through the lifestages: Importance for skin health and beauty. Plast. Aesthetic Res. 2021, 8,
2.
48. Yoneda, M.; Yamagata, M.; Suzuki, S.; Kimata, K. Hyaluronic acid modulates proliferation of mouse dermal fibroblasts in culture.
J. Cell Sci. 1988, 90 (Pt 2), 265–273.
49. Greco, R.M.; Iocono, J.A.; Ehrlich, H.P. Hyaluronic acid stimulates human fibroblast proliferation within a collagen matrix. J.
Cell. Physiol. 1998, 177, 465–473.
50. Röck, K.; Fischer, K.; Fischer, J.W. Hyaluronan used for intradermal injections is incorporated into the pericellular matrix and
promotes proliferation in human skin fibroblasts in vitro. Dermatology 2010, 221, 219–228.
51. Ciccone, V.; Zazzetta, M.; Morbidelli, L. Comparison of the Effect of Two Hyaluronic Acid Preparations on Fibroblast and Endothelial
Cell Functions Related to Angiogenesis. Cells 2019, 8, 1479.
52. Cabral, L.R.B.; Teixeira, L.N.; Gimenez, R.P.; Demasi, A.P.D.; de Brito Junior, R.B.; de Araújo, V.C.; Martinez, E.F. Effect of Hyaluronic
Acid and Poly-L-Lactic Acid Dermal Fillers on Collagen Synthesis: An in vitro and in vivo Study. Clin. Cosmet. Investig.
Dermatol. 2020, 13, 701–710.
53. Yevdokimova, N.Y.; Podpryatov, S.E. Hyaluronic acid production and CD44 expression in cultured dermal fibroblasts of patients
with non-insulin-dependent diabetes mellitus with and without chronic ulcers on the lower extremity. Wound Repair
Regen. 2005, 13, 181–188.
54. Wu, P.T.; Kuo, L.C.; Su, F.C.; Chen, S.Y.; Hsu, T.I.; Li, C.Y.; Tsai, K.J.; Jou, I.M. High-molecular-weight hyaluronic acid attenuated
matrix metalloproteinase-1 and -3 expression via CD44 in tendinopathy. Sci. Rep. 2017, 7, 40840.
Polymers 2023, 15, 2438 13 of 13
55. Pasonen-Seppänen, S.; Hyttinen, J.M.; Rilla, K.; Jokela, T.; Noble, P.W.; Tammi, M.; Tammi, R. Role of CD44 in the organization
of keratinocyte pericellular hyaluronan. Histochem. Cell Biol. 2012, 137, 107–120.
56. Bourguignon, L.Y. Matrix hyaluronan-activated CD44 signaling promotes keratinocyte activities and improves abnormal epidermal
functions. Am. J. Pathol. 2014, 184, 1912–1919.
57. Bourguignon, L.Y.; Ramez, M.; Gilad, E.; Singleton, P.A.; Man, M.Q.; Crumrine, D.A.; Elias, P.M.; Feingold, K.R. Hyaluronan-
CD44 interaction stimulates keratinocyte differentiation, lamellar body formation/secretion, and permeability barrier homeostasis.
J. Investig. Dermatol. 2006, 126, 1356–1365.
58. Herbold, K.W.; Zhou, J.; Haggerty, J.G.; Milstone, L.M. CD44 expression on epidermal melanocytes. J. Investig. Dermatol. 1996,
106, 1230–1235.
59. Schneider, M.; Rother, S.; Möller, S.; Schnabelrauch, M.; Scharnweber, D.; Simon, J.C.; Hintze, V.; Savkovic, V. Sulfated hyaluronan-
containing artificial extracellular matrices promote proliferation of keratinocytes and melanotic phenotype of melanocytes
from the outer root sheath of hair follicles. J. Biomed. Mater. Res. Part A 2019, 107, 1640–1653.
60. Takabe, P.; Kärnä, R.; Rauhala, L.; Tammi, M.; Tammi, R.; Pasonen-Seppänen, S. Melanocyte Hyaluronan Coat Fragmentation
Enhances the UVB-Induced TLR-4 Receptor Signaling and Expression of Proinflammatory Mediators IL6, IL8, CXCL1, and
CXCL10 via NF-κB Activation. J. Investig. Dermatol. 2019, 139, 1993–2003.
61. Borg, M.; Brincat, S.; Camilleri, G.; Schembri-Wismayer, P.; Brincat, M.; Calleja-Agius, J. The role of cytokines in skin aging.
Climacteric 2013, 16, 514–521.
62. Hu, L.; Nomura, S.; Sato, Y.; Takagi, K.; Ishii, T.; Honma, Y.; Watanabe, K.; Mizukami, Y.; Muto, J. Anti-inflammatory effects of
differential molecular weight Hyaluronic acids on UVB-induced calprotectin-mediated keratinocyte inflammation. J. Dermatol.
Sci. 2022, 107, 24–31.
63. Sörgel, C.A.; Schmid, R.; Stadelmann, N.; Weisbach, V.; Distel, L.; Horch, R.E.; Kengelbach-Weigand, A. IGF-I and Hyaluronic
Acid Mitigate the Negative Effect of Irradiation on Human Skin Keratinocytes. Cancers 2022, 14, 588.
64. Boeckx, R.L.; Dakshinamurti, K. Biotin-mediated protein biosynthesis. Biochem. J. 1974, 140, 549–556.
65. Chalifour, L.E.; Dakshinamurti, K. The biotin requirement of human fibroblasts in culture. Biochem. Biophys. Res. Commun. 1982,
104, 1047–1053.
66. Mock, D.M. Evidence for a pathogenic role of omega 6 polyunsaturated fatty acid in the cutaneous manifestations of biotin
deficiency. J. Pediatr. Gastroenterol. Nutr. 1990, 10, 222–229.
67. Proud, V.K.; Rizzo, W.B.; Patterson, J.W.; Heard, G.S.; Wolf, B. Fatty acid alterations and carboxylase deficiencies in the skin of
biotin-deficient rats. Am. J. Clin. Nutr. 1990, 51, 853–858.
68. Ogawa, Y.; Kinoshita, M.; Sato, T.; Shimada, S.; Kawamura, T. Biotin Is Required for the Zinc Homeostasis in the Skin. Nutrients
2019, 11, 919.
69. Pullar, J.M.; Carr, A.C.; Vissers, M.C.M. The Roles of Vitamin C in Skin Health. Nutrients 2017, 9, 866.
70. Hämäläinen, L.; Kärkkäinen, E.; Takabe, P.; Rauhala, L.; Bart, G.; Kärnä, R.; Pasonen-Seppänen, S.; Oikari, S.; Tammi, M.I.;
Tammi, R.H. Hyaluronan metabolism enhanced during epidermal differentiation is suppressed by vitamin C. Br. J. Dermatol.
2018, 179, 651–661.
71. Miao, F.; Su, M.Y.; Jiang, S.; Luo, L.F.; Shi, Y.; Lei, T.C. Intramelanocytic Acidification Plays a Role in the Antimelanogenic and
Antioxidative Properties of Vitamin C and Its Derivatives. Oxidative Med. Cell. Longev. 2019, 2019, 2084805.
72. Taira, N.; Katsuyama, Y.; Yoshioka, M.; Muraoka, O.; Morikawa, T. Structural Requirements of Alkylglyceryl-l-Ascorbic Acid
Derivatives for Melanogenesis Inhibitory Activity. Int. J. Mol. Sci. 2018, 19, 1144.
73. Solano, F. Metabolism and Functions of Amino Acids in the Skin. Adv. Exp. Med. Biol. 2020, 1265, 187–199.
74. Lu, Y.; Tonissen, K.F.; Di Trapani, G. Modulating skin colour: Role of the thioredoxin and glutathione systems in regulating
melanogenesis. Biosci. Rep. 2021, 41, BSR20210427.
75. Arjinpathana, N.; Asawanonda, P. Glutathione as an oral whitening agent: A randomized, double-blind, placebo-controlled
study. J. Dermatol. Treat. 2012, 23, 97–102.
76. Watanabe, F.; Hashizume, E.; Chan, G.P.; Kamimura, A. Skin-whitening and skin-condition-improving effects of topical oxidized
glutathione: A double-blind and placebo-controlled clinical trial in healthy women. Clin. Cosmet. Investig. Dermatol. 2014,
7, 267–274.
77. Weschawalit, S.; Thongthip, S.; Phutrakool, P.; Asawanonda, P. Glutathione and its antiaging and antimelanogenic effects. Clin.
Cosmet. Investig. Dermatol. 2017, 10, 147–153.
78. Sabbagh, F.; Kim, B.S. Recent advances in polymeric transdermal drug delivery systems. J. Control Release. 2022, 341, 132–146.
Disclaimer/Publisher’s Note: The statements, opinions and data contained in all publications are solely those of the individual author(
s) and contributor(s) and not of MDPI and/or the editor(s). MDPI and/or the editor(s) disclaim responsibility for any injury to
people or property resulting from any ideas, methods, instructions or products referred to in the content.
——————————
Citation: Siquier-Dameto, G.;
Boisnic, S.; Boadas-Vaello, P.; Verdú,
E. Anti-Aging and Depigmentation
Effect of a Hyaluronic Acid
Mechanically Stabilized Complex on
Human Skin Explants. Polymers
2023, 15, 2438. https://doi.org/
10.3390/polym15112438
Academic Editor: Alina Sionkowska
Received: 3 May 2023
Revised: 22 May 2023
Accepted: 23 May 2023
Published: 24 May 2023
Copyright: © 2023 by the authors. Licensee
MDPI, Basel, Switzerland.
This article is an open access article
distributed under the terms and conditions
of the Creative Commons Attribution
(CC BY) license (https://creativecommons.
org/licenses/by/4.0/).
Related Articles
-
Issue 15: Journal of Cosmetic Dermatology: Skin Wound Healing Following Injecting Hyaluronic Acid Rejuvenating Complex
Skin Wound Healing Following Injecting Hyaluronic Acid Rejuvenating Complex, Polycaprolactone, or Combination Therapy: An Experimental Study Noury Adel, Nenad Stankovic, Gerardo Cervantes, Amira…
Read Article -
Issue 14: AMWC 2025 RESEARCH POSTER: Combining PLLA with treatments containing stabilized HA, vitamin C and L-Carnitine has been shown to improve skin rejuvenation outcomes through a synergistic approach
Click on this link to read the PDF version of the Poster
Read Article -
Issue 13: IMCAS 2025 RESEARCH POSTER: L-Carnitine Subcutaneous Injection After HIFU for Local Fat Reduction: a Case Study
Click on this link to read the PDF version of the Poster
Read Article